Impact statement
The development and maintenance of reef-fronted tropical shorelines and reef islands are strongly dependent upon the supply of biogenic sediments produced on the adjacent coral reefs. It is well established that a wide array of reef-dwelling plants and animals either directly (e.g., in the form of their shelly remains) or indirectly (e.g., as a by-product of their feeding) contribute to the production of this sedimentary material. However, we lack standardised methodologies that can enable estimates of both the amounts and types of sediment being generated and the sediment size-class fractions to which this material contributes. Sediment composition and grain size are both important controls on shoreline sediment supply because they impact sediment hydrodynamic behaviour. Reliable estimates of production are thus needed to better support shoreline sediment transport and supply modelling. Here we introduce a new census-based method called SedBudget that has been set up to address this challenge. Based on a proposed set of consistent taxa-specific methodologies it generates estimates of total sediment production from reef taxa, and the types and size-class fractions of sediment generated. We then apply this data to several reef sites in the northern Chagos Archipelago to illustrate the nature of the data produced. Such data not only have potential to support coastal sediment transport modelling studies through the acquisition of site-level production estimates, but also to contribute to more integrated datasets capturing total site-level biogenic carbonate production that can encompass both reef framework and sediment production sides of this challenge. Further underpinning metrics and refinements of this initial iteration of SedBudget will improve its accuracy, and versions for use in other regions are currently under development, but we hope this article will in part stimulate additional research on this key topic.
Introduction
Biogenically-derived calcium carbonate is a defining feature of tropical coral reefs, and this carbonate accumulates both as reef framework (remnant coral skeletons being a primary contributor) and as detrital sediment. Our understanding of the carbonate production and erosion processes that underpin reef framework accumulation has advanced significantly over recent years (Browne et al., Reference Browne, Cuttler, Moon, Morgan, Ross, Castro‐Sanguino, Kennedy, Harris, Barnes, Bauman, Beetham, Bonesso, Bozec, Cornwall, Dee, TM, D’Olivio, Doropoulos, Evans, Eyre, Gatenby, González-Rivero, Hamylton, Hansen, Lowe, Mallela, O’Leary, Roff, Saunders and Zweifler2021) and supported the use of carbonate budget data as a metric for quantifying the geo-ecological functional ‘health’ of reefs (Mace et al., Reference Mace, Reyers, Alkemade, Biggs, Chapin, Cornell, Díaz, Jennings, Leadley, Mumby, Purvis, Scholes, Seddon, Solan, Steffen and Woodward2014; Perry and Alvarez-Filip, Reference Perry and Alvarez-Filip2019). In contrast, data on the production of reef-derived sedimentary carbonate (limited herein to material <64 mm in size, that is, pebble grade or below based on the Udden-Wentworth nomenclature), remains relatively sparse. Sedimentary carbonate is important because it contributes to the accumulating structure of reefs and lagoons (often more than 50% of a reef’s internal structure is sediment; Hubbard et al., Reference Hubbard, Burke and Gill1998) and to the formation of proximal sedimentary landforms such as beaches and reef islands (Perry et al., Reference Perry, Kench, Smithers, Riegl, Yamano and O’Leary2011a). Furthermore, because most reef sediment production is ecologically influenced, changes in reef ecology may bring about major shifts in the sedimentary carbonate production side of reef budgets (Perry and Alvarez-Filip, Reference Perry and Alvarez-Filip2019). Clearly, reef ecology has naturally fluctuated in response to past climatic forcings and other disturbances, but the speed of recent human-driven ecological change on reefs appears unprecedented. This is raising awareness of the need to improve our capacity for quantifying the amount, type and grain size of reef-derived sediments to support coastal vulnerability studies.
The primary sources of the biogenic sediment produced in reef settings are well known (Figure 1). Direct contributions derive post-mortem from skeletal fauna such as molluscs and foraminifera (Bosence, Reference Bosence1989), and from the disaggregation of the calcified segments of calcareous green and red algae (Neumann and Land, Reference Neumann and Land1975). The grazing activities of some species of parrotfish and sea urchins, which excrete carbonate particles after ingestion (Hunter, Reference Hunter1977; Bellwood, Reference Bellwood1996), also represent a major sediment source, and species of triggerfish and pufferfish can produce coral rubble as they break coral colonies whilst foraging (Perry et al., Reference Perry, Salter, Lange, Kochan, Harborne and Graham2022). All bony marine fish (teleosts) additionally excrete silt and clay-grade carbonate generated through intestinal secretions (Perry et al., Reference Perry, Salter, Harborne, Crowley, Jelks and Wilson2011b; Salter et al., Reference Salter, Perry and Wilson2012). Many seagrasses also indirectly contribute sedimentary carbonate in the form of the calcareous epiphytes that colonise seagrass blades (Corlett and Jones, Reference Corlett and Jones2007). The generation of larger sedimentary clasts, known as coral rubble (typically >64 mm in size, i.e., cobble and boulder grade), is a common product from physical disturbances (Scoffin, Reference Scoffin1992, Reference Scoffin1993).

Figure 1. Schematic showing the main sources (bold text at top) of biogenically-derived sediment in coral reef habitats, and the main resultant sediment constituent types that arise from these sources (in italics at bottom).
There is thus a comprehensive understanding of reef-derived sediment sources and of the processes that indirectly, for example, as a by-product of feeding, generate sediment. Furthermore, it is recognised that as a function of differing ecologies, reef sediment sources will vary within and between reef systems, with resultant divergent implications for reef accretion and landform sediment supply (Perry et al., Reference Perry, Kench, Smithers, Riegl, Yamano and O’Leary2011a, ). Various past studies have sought to quantify these inputs, often for individual groups of producers, for example, for foraminifera (Hallock-Muller, Reference Hallock-Muller1974; Fujita et al., Reference Fujita, Osawa, Kayanne, Ide and Yamano2008; Doo et al., Reference Doo, Hamylton and Bryne2012), calcareous green algae (Neumann and Land, Reference Neumann and Land1975; Multer, Reference Multer1988), and seagrass epiphytes (Nelsen and Ginsburg, Reference Nelsen and Ginsburg1986), and occasionally for wider suites of producers (e.g., Bosence et al., Reference Bosence, Rowlands and Quine1985; Browne et al., Reference Browne, Smithers and Perry2013; Perry et al., Reference Perry, Morgan and Yarlett2017). Given the increasing knowledge of, and interest in, reef sediment generation in the context of reef carbonate budget studies (Browne et al., Reference Browne, Cuttler, Moon, Morgan, Ross, Castro‐Sanguino, Kennedy, Harris, Barnes, Bauman, Beetham, Bonesso, Bozec, Cornwall, Dee, TM, D’Olivio, Doropoulos, Evans, Eyre, Gatenby, González-Rivero, Hamylton, Hansen, Lowe, Mallela, O’Leary, Roff, Saunders and Zweifler2021), and the development of different methodologies for estimating sediment production rates, there is now an opportunity to build on this knowledge base and start developing a standardised methodology that can be applied across reef settings.
Here we present a methodology (SedBudget) that uses a census-based approach, comparable to that utilised in ReefBudget for framework carbonate budget studies (Perry et al., Reference Perry, Edinger, Kench, Murphy, Smithers, Steneck and Mumby2012, Reference Perry, Lange and Januchowski-Hartley2018), that is aimed at supporting estimates of sediment generation from a wide range of biogenic sources. This includes estimates of carbonate production rates (kg CaCO3 m−2 yr−1, hereafter described by the term G), the grain-size fractions of carbonate generated, and the contributions to different sediment constituent classes. We illustrate the use of SedBudget by applying it to shallow ocean- and lagoonward reefs around two islands in the Chagos Archipelago, Indian Ocean. We make openly available online a methodology handbook and the supporting data entry spreadsheets. This initial iteration of SedBudget should be seen as a first step to developing this important field of enquiry, and so we highlight those taxa for which methodological developments/refinements are most urgently needed. SedBudget has potential for application on a stand-alone basis for supporting reef-shoreline sediment linkage studies, to help parameterise coastal vulnerability (e.g., Storlazzi et al., Reference Storlazzi, Elias, Field and Presto2011) and reef-to-island sediment models (Morgan and Kench, Reference Morgan and Kench2014; Bramante et al., Reference Bramante, Ashton, Storlazzi, Cheriton and Donnelly2020; Masselink et al., Reference Masselink, Beetham and Kench2020), and/or to be used alongside ReefBudget for more complete biogenic carbonate budget assessments.
‘SedBudget’: Current scope and field methodologies
Our initial focus in developing SedBudget has been for its application to sites in the central-western Indian Ocean (IO), and here we describe the methodology as it relates to those biogenic sediment sources previously shown to be most important within shallow reef, beach and island systems in this region. Whilst the relative abundance of specific sedimentary constituents inevitably varies between locations and habitats (Gischler, Reference Gischler2006), the major sediment constituents in the <64 mm size classes (i.e., pebble grade and below) in reef-island sediments in this region have, in typically decreasing order of abundance, been shown to be coral, crustose coralline algae (CCA), Halimeda, molluscs (bivalves and gastropods), and benthic foraminifera, with echinoid spines a generally minor component (Perry et al., Reference Perry, Kench, O’Leary, Morgan and Januchowski-Hartley2015; East et al., Reference East, Perry, Kench and Liang2016; Jorry et al., Reference Jorry, Camoin, Jouet, Roy, Vella, Courgeon, Prat, Fontanier, Paumard, Boulle, Caline and Borgomano2016). Coral and CCA are mainly derived from the feeding activities of parrotfishes and sea urchins (Hunter, Reference Hunter1977; Yarlett et al., Reference Yarlett, Perry and Wilson2021). In some locations in the eastern IO region, high numbers of molluscs occur in reef and island sediments (Bonesso et al., Reference Bonesso, Browne, Murley, Dee, Cuttler, Paumard, Benson and O’Leary2022), and around Mauritius (south-west IO) high foraminifera and mollusc densities have been noted (Karisiddaiah et al., Reference Karisiddaiah, Veerayya and Guptha1988). It is this suite of regionally relevant taxa, and the processes that indirectly or directly contribute to the production of these sediment constituents, that we primarily focus on here. Additionally, we include methodologies for estimating sediment production from other fish groups (triggerfishes and pufferfishes), from other calcareous green algae known to occur in the region (specifically Penicillus spp. and Udotea spp.), and from seagrass epiphytes.
The field data collection approach in SedBudget is broadly similar to that used by ReefBudget (Perry et al., Reference Perry, Edinger, Kench, Murphy, Smithers, Steneck and Mumby2012, Reference Perry, Lange and Januchowski-Hartley2018; Perry and Lange, Reference Perry and Lange2019) in that it is census-based and user-adaptable for different sites or regions (a Caribbean-specific version is currently in development). As developed, the methodology involves benthic data collection within replicate (0.5 × 0.5 m) quadrats placed at 1-m intervals along 10 m long transects within a given study habitat (Figure 2), and fish census data collected from replicate belt transects in near proximity to the benthic data collection. This means that both ReefBudget and SedBudget can be conducted along the same transects, located as deemed appropriate to the individual study site. This census-based approach is used as the basis for collecting data on the abundance of each sediment producer of interest.

Figure 2. Summary cartoon showing approach to surveys, equipment and a basic step-by-step to the data collection (inset shows quadrat and spacing of profile survey lines). Quadrats can be placed at intervals either along the same transect side (as shown in the figure) or in a checkerboard style if deemed more appropriate to the site.
Calculations of total sediment generation (G) from different taxa and processes and the contributions of this sediment to different grain-size classes are all made within the online supporting data entry spreadsheets (see: https://geography.exeter.ac.uk/sedbudget/). Tabs containing known available metrics that underpin the sediment production calculations for each producer are included within the spreadsheets. At present the method only provides estimates of sediment generation associated with either the release of carbonate as a by-product of bioerosion, or that associated with the post-mortem inputs of skeletal material. Inputs associated with physical framework damage (coral rubble generation, etc.) cannot yet be accounted for, although these primarily contribute to clasts larger than the upper size class boundary considered here (i.e., >64 mm). The basic approaches to data collection are outlined below, first for the fish and then for the benthic contributors. Further, it is recommended to conduct fish surveys first to limit any disturbance to these communities during benthic surveying. Additional data entry instructions are provided in the online methodology handbook and spreadsheets and should be referred to alongside the descriptive text below. All the underpinning metrics are user-adaptable to allow the usage of species or site-specific appropriate data.
Estimating sediment generation by parrotfish
Estimates of sediment generation by parrotfishes in SedBudget are based on the same underlying approaches used for estimating substrate erosion in ReefBudget (Perry et al., Reference Perry, Lange and Januchowski-Hartley2018). The rationale for this is that there is no evidence of intestinal dissolution of ingested reef substrates in the guts of parrotfishes, and so bioerosion rate can be taken as a proxy for sediment generation (Perry et al., Reference Perry, Morgan, Lange and Yarlett2020). A site-specific census approach using belt transects (suggested size 30 × 5 m, n = 4–6 transects per habitat) and located close to the benthic transects allows the collection of parrotfish species, life phase and size class data. This data is then entered into the SedBudget fish census sheet, and estimates of parrotfish-derived sediment generation calculated based on published data on bite rates, proportions of bites leaving scars, and scar volumes by species and fish body size. There now exists such data for a quite wide range of IO parrotfish species (SI Table in Lange et al., Reference Lange, Perry and Alvarez-Filip2020; and ReefBudget website at https://geography.exeter.ac.uk/reefbudget/for%20latest%20updates).
In a sediment generation context, it is also necessary to factor for the proportions of sediment produced in different grain-size classes. For this, SedBudget makes use of existing published data on the sediment grain-size fractions in excreted carbonate determined for several common IO and Indo-Pacific (IP) Scarus spp. and Chlorurus spp. (Hoey and Bellwood, Reference Hoey and Bellwood2008; Yarlett et al., Reference Yarlett, Perry and Wilson2021). Sister-species or closely functionally related species (Choat et al., Reference Choat, Klanten, Van Herwerden, Robertson and Clements2012) or mean genera-level data are necessarily applied to species where no grain-size data currently exists. An important additional aspect of the release of sedimentary carbonate from parrotfishes is that the composition of the excreted faecal material reflects the substrates on which parrotfishes feed. This is mostly coral, but not entirely, and to account for this, SedBudget integrates published data on the composition of the material excreted by a range of species and size classes of IO parrotfishes – this work showing that ~95% of the excreted carbonate is coral, and the remaining material mostly CCA (Yarlett et al., Reference Yarlett, Perry and Wilson2021). Resultant outputs thus provide an estimate of total sediment generation (G) and the mean proportional contributions to different grain-size classes and sediment constituents.
Estimating sediment generation by triggerfish and pufferfish
Species of both pufferfishes and triggerfishes can generate coarse sand to pebble grade sedimentary material through physical breakage of reef framework (Guzman and Lopez, Reference Guzman and Lopez1991; Glynn and Manzello, Reference Glynn and Manzello2015), either whilst feeding on coral (some pufferfishes) or whilst foraging for invertebrates in coral skeletons (some triggerfishes) (Perry et al., Reference Perry, Salter, Lange, Kochan, Harborne and Graham2022). Estimates of sediment generation by triggerfishes and pufferfishes in SedBudget are thus based on similar conceptual approaches that inform estimates for parrotfishes, that is, sediment generation rates for fish of a given species and size class, and the size-class fractions of sediment generated. In the field, data on the numbers of trigger- and pufferfishes and their size are collected in belt transects together with parrotfish data, with estimates of sediment generation based on species-specific erosion rate data and on the sizes of material generated. For both groups, it is important to note that rate data is presently sparse, little account can be taken for fish of different sizes, and sediment grain-size data is qualitative not quantitative (Glynn et al., Reference Glynn, Stewart and McCosker1972; Alvarado et al., Reference Alvarado, Grassian, Cantera-Kintz, Carballo, Londoño-Cruz, Glynn, Manzello and Enochs2017). What data does exist is used here but clearly caution must for now be exercised in the interpretation of resultant rate data where these fish are present in high abundances.
Substrate metrics and benthic composition data
The first stage of the benthic assessments is to establish a set of 10-m transects (see Figure 2). Each quadrat along a transect line is initially examined to quantify the relative %-cover of the following major substrate categories; live coral, dead coral w/ CCA; dead coral w/ turf; macroalgae; coral rubble; sand and seagrass. This can either be done visually with the aid of a %-cover estimator chart, or post-surveying by point counting quadrat images. In addition, an estimate of substrate rugosity is made by measuring the full surface profile at three points across each quadrat using either a flexible tape or chain with known segment lengths (Risk, Reference Risk1972; Figure 2). These quadrat-level %-cover estimates and profile measurements are then entered into the ‘Substrate’ tab of the spreadsheet from which mean %-cover and rugosity metrics are calculated and are automatically pulled across into the sediment production sheets of the different constituents where relevant to subsequent production estimates.
Estimating sediment generation by sea urchins
Estimates of sediment generation by sea urchins are also based on the underlying principles used in ReefBudget, utilising survey data on urchin species and test sizes per unit area of reef. These data are integrated with published erosion rates for different urchin species and test sizes within species (e.g., Bak, Reference Bak1990; Griffin et al., Reference Griffin, Garcia and Weil2003; Alvarado et al., Reference Alvarado, Cortés, Guzman and Reyes-Bonilla2016). Again, there is no evidence to suggest that intestinal dissolution of ingested reef substrates takes place and so bioerosion rate can be taken as a proxy for sediment generation. In the field, species and test size of every urchin are recorded per quadrat, with existing species-specific test size-erosion rate relationships used to estimate total sediment generation rates. As for parrotfishes, there is a further need to consider the proportion of sediment produced in different grain-size classes. Some data on this exists for different test-size specimens of Echinometra mathaei from IP locations (Chazottes et al., Reference Chazottes, Chevillotte and Dufresne2004) and for a range of test-size classes of Diadema antillarum and for small Echinometra lucunter collected from the Bahamas (Hale et al., in preparation – but see data entry spreadsheet for summary of approach used). The spreadsheet system applies these data to the relevant species, or to similar species, to then provide current best estimates of the proportion of sediment generated in different grain-size classes. A further aspect of the release of sedimentary carbonate in excreted faecal material relates to the substrates on which urchins feed. Few studies have examined this, but data for D. antillarum (Hunter, Reference Hunter1977) and E. mathaei (Chazottes et al., Reference Chazottes, Chevillotte and Dufresne2004) are used to estimate the proportional contributions of different grain types (mostly coral and CCA) from urchin erosion.
Estimating sediment generation by endolithic sponges
Various studies using experimental substrates have quantified endolithic sponge erosion (e.g., Tribollet and Golubic, Reference Tribollet and Golubic2005; Carreiro-Silva and McClanahan, Reference Carreiro-Silva and McClanahan2012). Whilst such studies showed that sponge erosion can be a significant contributor to carbonate erosion, from a sedimentary perspective the process is also important because it generates fine-grained ‘chips’ of expelled sediment (mostly <0.1 mm in size; Fütterer, Reference Fütterer1974). However, because the erosion process involves chemical dissolution, sponge bioerosion rates do not directly equate to a measure of sediment generation. The proportion of eroded substrate lost to dissolution during chamber excavation needs to be factored for (the difference then being the remaining solid material that is expelled as sediment). Limited data on this process exists, but on average ~ 38% of sponge-eroded material is lost to dissolution (Zundelevich et al., Reference Zundelevich, Lazar and Ilan2007; Nava and Carballo, Reference Nava and Carballo2008). This value is used in SedBudget, alongside published sponge erosion rate data and the proportion of available erodible substrate per unit area, to estimate sponge-derived sediment generation rates. Here users need to make an informed decision about which of any available rate data they deem appropriate for their study location. SedBudget then also factors for the contribution of this material to different sediment grain-size fractions based on data from Fütterer (Reference Fütterer1974) and de Bakker (in preparation – but see data entry spreadsheet for summary of approach used) for a range of endolithic sponge species.
Estimating sediment generation by calcareous green algae
Numerous species of several genera of calcareous green algae including Halimeda, Penicillus, Udotea and Rhipocephalus are known contributors to sediment generation in the form of their calcified plant segments (Neumann and Land, Reference Neumann and Land1975). Of these, species of the first three genera listed occur in the IO region or are pan-tropically distributed, and methods for estimating sediment generation by all three genera are included. Previous approaches for estimating carbonate production by calcifying green algae have involved either sampling plants from known unit areas of reef and estimating plant segment production, and/or assessments of plant abundance and carbonate content (Neumann and Land, Reference Neumann and Land1975; Drew, Reference Drew1983; Multer, Reference Multer1988; Payri, Reference Payri1988). In developing SedBudget, our aim has been to limit destructive sampling where possible, accepting that localised sampling may be needed to provide metrics supporting sediment generation estimates, for example, on plant/segment carbonate content and on plant turnover rates. We thus use a method adapted from that proposed by Freile (Reference Freile2004) based on data on Halimeda spp. plant abundance, plant carbonate content, and plant turnover rates. This approach has been previously modified to support carbonate sediment production estimates for several Halimeda spp. (Perry et al., Reference Perry, Morgan and Salter2016) and then expanded to include other green algal species, including Penicillus spp. and Udotea spp. (Perry et al., Reference Perry, Salter, Morgan and Harborne2019). The approach uses species-specific relationships between plant biovolumes (as used to quantify biovolumes of corals; Naumann et al., Reference Naumann, Niggl, Laforsch, Glaser and Wild2009) and the carbonate content of plants of different sizes. The volume of each plant in a quadrat is determined from in-field measured plant dimensions and used to estimate the carbonate content of each plant. This data is then combined with published or locally collected crop-per-year data to estimate annual carbonate sediment production rates. In addition, post-mortem plant breakdown data can be applied to estimate proportional contributions to different sediment grain-size classes (based on data in Perry et al., Reference Perry, Morgan and Salter2016, Reference Perry, Salter, Morgan and Harborne2019). Known existing metrics relevant to the above calculations are provided in the supporting metrics tabs for each producer group, but where specific metrics cannot be collected locally and appropriate data has not yet been published, we would recommend using an average of existing data grouped by growth form.
Estimating sediment generation by bivalves and gastropods
Bivalves and gastropods represent significant sedimentary carbonate producers in some reef habitats (Karisiddaiah et al., Reference Karisiddaiah, Veerayya and Guptha1988) and often especially in those that are sediment-dominated as many molluscs live within the sediment (e.g., Browne et al., Reference Browne, Smithers and Perry2013). Estimating sediment generation rates by bivalves and gastropods are made complex by the fact that species-level annual production rate data are limited. SedBudget thus presently makes use of data on annual carbonate production rates per individual summarised in Bosence (Reference Bosence1989). Clearly, however, until additional data on production rates per individual becomes available caution should be exercised in the interpretation of resultant rate data on this producer group. This caveat aside, in the field, the number of living infaunal and epifaunal bivalves and gastropods per quadrat are counted and the maximum size of each specimen is recorded. In framework-dominated habitats, with only a thin sediment veneer, counts for the entire quadrat may be feasible. In sediment-dominated habitats where the sediment depth exceeds a few cm, it may be more appropriate to extract sediment samples from a known area to a depth of ~10 cm and to then isolate specimens in water using a small hand-held sieve (mesh size 2 mm). Infaunal bivalves are then counted, measured (all shells >4 mm are classed together) and the numbers scaled to the quadrat area. Total sediment production rates for both bivalves and gastropods are then estimated and the proportion of shells in different size-class fractions used to determine grain-size contributions, with the necessary current assumption that shell size reflects sediment input size. Note that the post-mortem diminution of molluscan shells by fish feeding (e.g., that associated with feeding by tuskfish; Nilsen et al., Reference Nilsen, Bonesso, Benson, Dee, Browne, Morgan, O’Leary, Cuttler and McIlwain2022) or through physical breakdown processes are not yet readily factored for.
Estimating sediment generation by benthic foraminifera
Foraminifera are often major reef sediment producers. However, their generally small size and the challenges of measuring populations and test sizes in situ makes estimates of sediment production based on in-field data collection problematic. In this initial iteration of SedBudget, we thus adopt an approach proposed by Langer et al. (Reference Langer, Silk and Lipps1997), whereby foraminifera test production rates are estimated as a function of the proportion of tests counted in sediment samples collected from each transect, multiplied by a set productivity value depending on reef zone (reef framework and rubble habitats: 6 g m−2 yr−1; sand-dominated lagoon habitats: 1.2 g m−2 yr−1; Langer et al., Reference Langer, Silk and Lipps1997). This approach lacks detail on the taxa present and their grain-size contributions, the latter being necessary for consistency with the other methodologies in SedBudget. Our approach here is thus to integrate counts of foraminifera from size-fraction sieved samples such that the proportional contributions of foraminifera to size-class fractions can be calculated. This approach clearly only crudely accounts for site-relevant productivity rates and consequently, we are looking to develop a future alternative aligned to the ‘simple method’ (after Hallock, Reference Hallock1981) described by Narayan et al. (Reference Narayan, Reymond, Stuhr, Doo, Schmidt, Mann and Westphal2022), whereby carbonate production rate can be calculated based on the current standing crop of foraminifera and then factoring for family-level turnover rate and mass data for a given test size class (Narayan et al., Reference Narayan, Reymond, Stuhr, Doo, Schmidt, Mann and Westphal2022). Once refined, this alternative methodology will appear in a revised iteration of the SedBudget methodology.
Estimating sediment generation by seagrass epibionts
The calcareous epiphytes that colonise seagrass blades can make a locally important contribution to carbonate sediment production post-blade die-off (Corlett and Jones, Reference Corlett and Jones2007). The calcareous components of these epiphyte communities are typically dominated by coralline algae, foraminifera and, polychaete and serpulid worms. Several studies have quantified carbonate production rates of epiphyte communities on seagrass and showed that plant and blade density, and the amount of carbonate per blade, are important controls on resultant production (Nelsen and Ginsburg, Reference Nelsen and Ginsburg1986; Perry and Beavington-Penney, Reference Perry and Beavington-Penney2005; East et al., Reference East, Johnson, Perry, Finlay, Musthag, Zahir and Floyd2023). SedBudget uses data generated in these previous studies (specifically on epibiont carbonate content, crops per year and epibiont grain-size contributions), but these can be exchanged for locally determined rates following established methodologies (Nelsen and Ginsburg, Reference Nelsen and Ginsburg1986). By combining data on the amount of epiphytic carbonate on plant blades, with in-field survey data on plant abundance and published crop-per-year data, SedBudget provides an estimate of total sediment production rate and the proportional contributions to different size-class fractions.
Field application of the methodology: A case study from the Chagos Archipelago
Study area
To examine the application of SedBudget we used the described methodology to estimate taxa-level sediment production rates, and to calculate resultant grain constituent production and sediment grain-size class contributions at four sites (two oceanward, two lagoonward) around two islands (Île Anglaise and Île de la Passe) in the northern part of Salomon Atoll, Chagos Archipelago (Figure 3A,B). Surveys (n = 4 transects per site) were conducted in January 2022 along the ~2 m depth contour just seaward of the reef crest/flats on the oceanward side of both islands, and in water depths of ~1 m across the fringing reefs on the lagoonward side of both islands (Figure 3C,D) – our strategy being to focus on the shallowest sites feasible to work at because of their relevance to shoreline sediment supply. In keeping with the recommended approach of SedBudget we used local or region-specific underpinning metrics where possible, and we list these and necessary species substitutions in Table 1. Resultant production estimates were compared to data on the composition of the sediment accumulating on the reefs themselves, and on the lower and upper beachfaces proximal to each site. Sediment composition was quantified from the analysis of resin-embedded and thin-sectioned samples using the image analysis software JMicrovision (n = 300 grain counts per sample). These same sediment samples were also sieved to quantify proportional contributions to the same grain-size classes used in SedBudget.

Figure 3. (A) Map showing the location of Salomon Atoll within the Chagos Archipelago. Inset shows the regional setting of the Chagos Archipelago; (B) Oblique drone image south-west across Salomon Atoll showing the location of the two exemplar study islands used; (C) View northwards across Île de la Passe; (D) View north-west across Île Anglaise. Dots in (C) and (D) show the location of the lagoonward and oceanward survey sites. Drone images courtesy of Rob Dunbar.
Table 1. Summary of key metrics used to derive estimates of sediment generation rates, grain-size contributions and sediment composition at the study sites in the Salomon Atoll, Chagos Archipelago
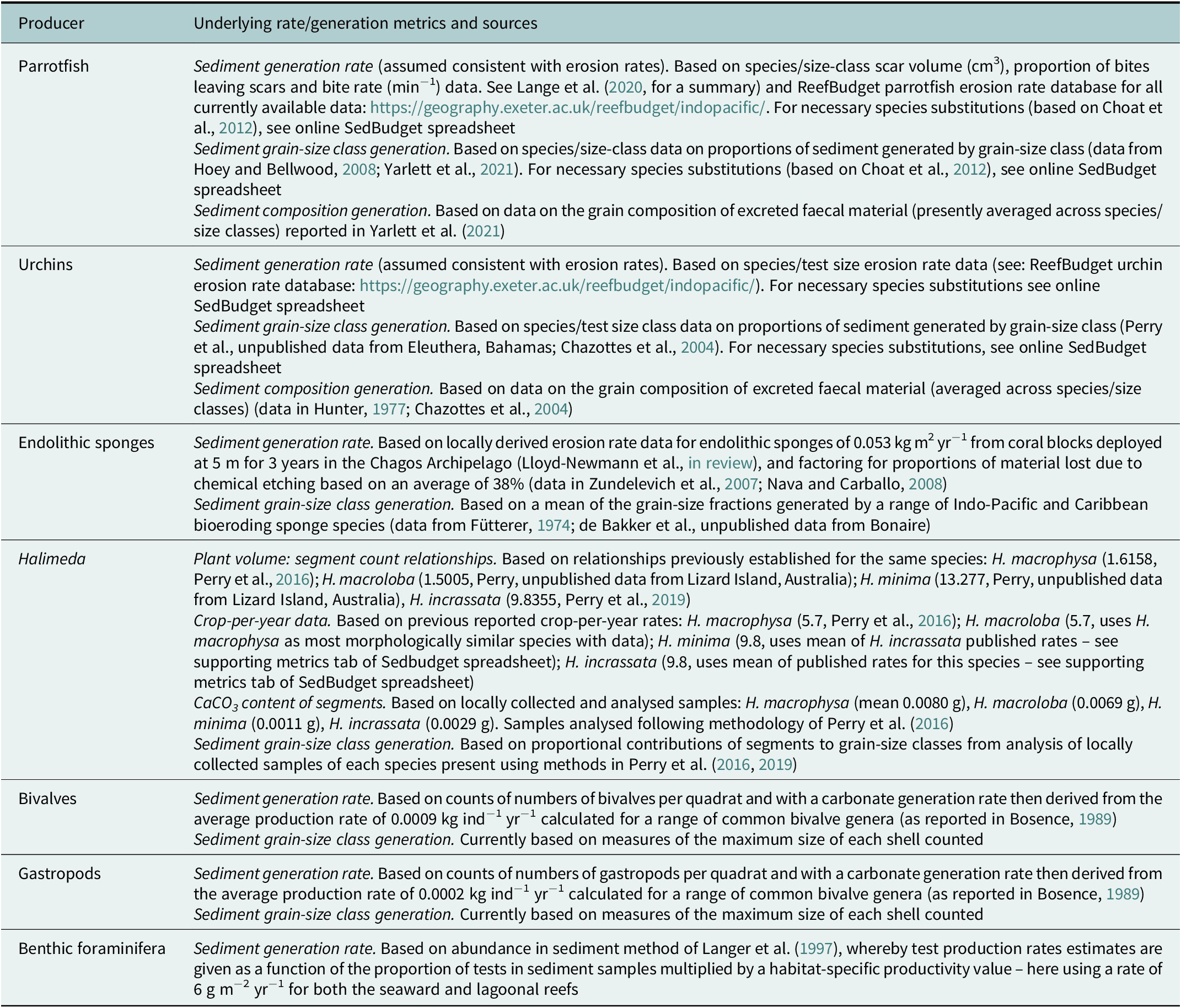
SedBudget-derived and in situ sedimentary data
SedBudget estimates of total sediment generation (G) across the four study sites ranged from (mean ± SE) 0.7 ± 0.1 G to 4.3 ± 1.3 G (Figure 4A and Supplementary Table S1). Production was dominated at all sites by sediment derived from parrotfishes (>90%), the exception being at the seaward Île de la Passe site where parrotfish abundance was lower and urchins contributed ~20% (Figure 4A). Varying secondary contributions across sites derived from endolithic sponges (~1–7%) and benthic foraminifera (~0.5–3.5%). Calculated proportional contributions from Halimeda spp. and molluscs were low (<0.5%). These taxa-level contributions result in the predicted generation of sediments that at all sites would be overwhelmingly coral-dominated (83–94%), and with the only common secondary constituent being CCA (range ~5–12%; Figure 4B), this resulting both from parrotfish but also urchin sediment generation. Foraminifera contribute up to a further ~3% of sediment, but all other constituents are rare. The SedBudget model also predicts that most of the sediment across these sites (65–75%) would be generated within the medium (0.25–0.5 mm) to very coarse sand (1–2 mm) size-class fractions (Figure 5E).

Figure 4. (A) Total estimated sediment production rates (mean ± SE, kg CaCO3 m−2 yr−1) at each site and the contributions made by different fish and benthic sediment producers. (B) Estimated proportions of different sediment constituents at each site resulting from fish and benthic sediment producers.
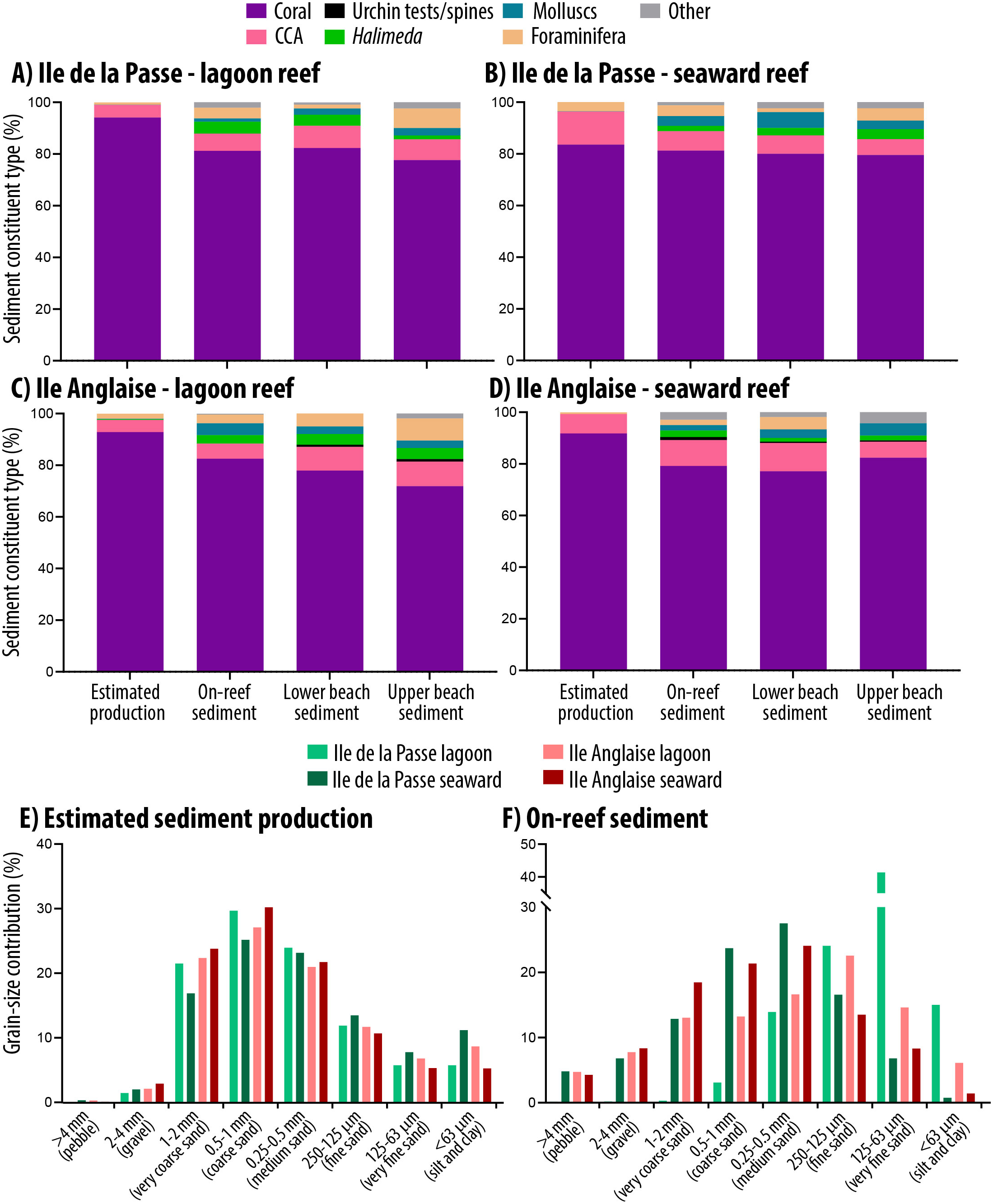
Figure 5. (A–D) Plots showing the relative proportions of sediment constituent types from SedBudget estimates (right-hand bar in each plot), compared to data based on grain constituent counts in proximal on-reef and lower and upper beachface sediment samples at each site. (E) Estimated contributions from SedBudget of sediment generated at each site by sediment grain-size class. (F) Measured contributions of sediment to each grain-size class based on sieve analysis within proximal on-reef sediment samples.
A comparison of the SedBudget estimates with sediment constituent and grain-size data from sediment samples from proximal on-reef (3 m depth in lagoonward, 5 m depth in oceanward reefs) and adjacent island beachface sites generally show a high degree of consistency. On-reef sediment samples are similarly coral-dominated (range: 79–82%) (Figure 5A–D and Supplementary Table S1), with CCA fragments the next most important constituent (range: 6–10%). All other constituents (foraminifera, Halimeda, molluscs and echinoid tests/spines) are relatively rare (Figure 5A–D), but slightly more abundant in the sediment than predicted by SedBudget. Lower beachface sediments are compositionally similar to the shallow reef sediments, whilst upper beachface samples often contain slightly greater proportions of molluscs, foraminifera and a small % of unidentified (micritised) grains (Figure 5A–D). Grain-size class data from the on-reef sediments is broadly similar to that predicted by SedBudget. Sediments are dominated by medium- to very coarse-sized sands (45–65%), except that there is overall an increased abundance of sediment in the larger grain-size classes, and at the Île de la Passe lagoonward reef high proportions of sediment occur in the fine and very fine sand-class fractions (Figure 5F).
Discussion
The current absence of a standardised methodology for assessing reef-derived sediment generation rates that can account for multiple contributing taxa represents a major gap. This is not only relevant in terms of better understanding wider reef carbonate production and cycling regimes (Browne et al., Reference Browne, Cuttler, Moon, Morgan, Ross, Castro‐Sanguino, Kennedy, Harris, Barnes, Bauman, Beetham, Bonesso, Bozec, Cornwall, Dee, TM, D’Olivio, Doropoulos, Evans, Eyre, Gatenby, González-Rivero, Hamylton, Hansen, Lowe, Mallela, O’Leary, Roff, Saunders and Zweifler2021), but also for applied reasons, that is, for quantifying and predicting shoreline sediment supply potential. This will be an area of growing importance given the likelihood of changes to reef-derived sediment supply under ecological reef degradation and sea-level rise (Bramante et al., Reference Bramante, Ashton, Storlazzi, Cheriton and Donnelly2020). Sustaining high-rate sediment supply to reef-proximal beach and island systems is not, however, simply dependent upon the total amount of sediment produced, but also the size-class fractions generated, because grain-size class and composition controls carbonate sediment hydrodynamic behaviour (de Kruijf et al., Reference de Kruijf, Slootman, de Boer and Reijmer2021). SedBudget has been set up to start to address these challenges. It generates estimates of not only overall sediment production rates, but also the size fractions of sediment produced as a function of the abundance and productivity of the major sediment-generating taxa at a site.
The application example we present from the Chagos Archipelago illustrates the nature of the data the methodology can generate. These data suggest that coral is the major sedimentary constituent being generated on both the oceanward and lagoonward reefs in this location, and that CCA is the next most common constituent (Figure 4). These findings are consistent with previous production estimates from the region (Perry et al., Reference Perry, Kench, O’Leary, Morgan and Januchowski-Hartley2015; Morgan and Kench, Reference Morgan and Kench2016) and reflect the relatively large numbers of parrotfish at all sites and of bioeroding urchins at the seaward sides of both islands. Differences between sites and reef position (lagoonward vs. oceanward) also largely reflect site-specific differences in parrotfish species abundance. Importantly, these findings are consistent with the constituent abundance data from proximal reef and island beach sediment samples which are also coral- and CCA-dominated (Figure 5). SedBudget-based constituent estimates do however identify slightly lower proportional contributions to the sediment by Halimeda (<0.3% at all sites), molluscs (<0.2%) and foraminifera (<3.5%) compared to proportions in both on-reef and proximal beach sediments (Figure 5). The island beach sediments will of course reflect the long-term averaging of sediments derived from multiple nearshore habitats and/or the effects of preferential sediment transport, and so some inherent differences should be expected. Plate-like Halimeda segments and bivalve shells, and gastropods and foraminifera tests are especially likely to be more easily physically transported to beach and nearshore zones because of their lower settling velocities or higher entrainment potential (Braithwaite, Reference Braithwaite1973; Kench and McLean, Reference Kench and McLean1996; de Kruijf et al., Reference de Kruijf, Slootman, de Boer and Reijmer2021). These grain characteristic-controlled transport influences will also to some extent influence resultant sediment grain-size distributions and probably partially contribute to the increased abundance of these constituent types observed (Figure 5). Physical breakage of dead coral skeletons, which could not be accounted for in this methodology, is also likely a contributing factor. One distinct grain-size difference observed is the skew towards finer-grained sediments in the Île de la Passe lagoon site (and to a lesser extent at the Île Anglaise lagoon site) compared to that predicted. The reasons for this are unclear but may reflect local hydrodynamic conditions favouring fine sediment deposition.
We also acknowledge that some of the differences observed between predicted and observed sediment compositions may be linked to the methodologies, available rate data or the local environmental history. For Halimeda, a key point is that we counted very few plants in surveys (mean max <4 plants m−2) and almost none at the oceanward sites. The higher abundance of Halimeda segments in adjacent on-reef sediment samples (up to 4%) thus probably reflects the previous increased abundance of Halimeda cover that occurred in these locations immediately post- the 2015/2016 bleaching event (Benkwitt et al., Reference Benkwitt, Wilson and Graham2019). That previous high Halimeda cover is no longer observed, but the legacy of that increase appears to persist in the reef sediments. It is relevant to note that in related work using the same methods on Halimeda production in the Maldives, where Halimeda cover was higher, that Halimeda were on average estimated to generated about 0.01 kg of sedimentary carbonate per % of cover (Perry et al., Reference Perry, Morgan and Salter2016, Reference Perry, Morgan, Lange and Yarlett2020). In locations with higher Halimeda cover, we would thus expect the method to generate higher relative contributions to overall sediment budgets. Benthic foraminifera abundances are also generally a little higher within on-reef sediments (2–4%) compared to SedBudget estimates (0.7–3.5%). This is potentially a function of the current methodology that necessarily uses average and not genera- or site-specific productivity values, and which one may imagine to be higher in these low-latitude locations.
Molluscan fragments similarly are markedly more abundant (by several %) in most sediment samples (Figure 5). Bosence (Reference Bosence1989) also noted a higher presence of molluscs in sediments in Florida Bay than predicted from production estimates. As in that study, we suggest this may partly reflect the challenges of mollusc sampling, but also the paucity of data on site-specific productivity rates that may be higher in our lower latitude locations compared to Florida Bay from where we have necessarily derived annual production rate data. These issues aside, the SedBudget-derived data seems to broadly reflect the composition and size class fractions of sediment that exist in the local sediment reservoirs, and the consistency between the two will hopefully improve as additional method adaptations and underpinning datasets emerge. It would however also be of interest to apply the method to a range of other types of reef locations where the relative abundance of producer groups are markedly different (e.g., sites with low grazer pressure) and to assess the sensitivity and data gaps to be addressed in the context of those types of reef settings.
As with its predecessor ReefBudget, SedBudget has been designed to be entirely user-adaptable such that the most appropriate, ideally locally collected, underpinning metrics can be utilised. However, as outlined, it is the case that some currently used approaches clearly need additional underpinning metrics to improve production-rate and grain-size estimates. For mobile substrate bioeroders that generate sedimentary carbonate as a by-product of feeding, for example, specific species of parrotfishes and urchins, relationships between body size (fish length or urchin test diameter) and erosion/sediment generation rate exist. Additional data in these areas is always beneficial, but would be most useful for a wider range of ‘excavating’ (i.e., high rate eroder/sediment generating) parrotfish species in order to limit necessary species substitutions. Feeding and erosion rate data from a wider range of higher latitude or more marginal reef settings would also be useful. For triggerfishes and pufferfishes there is a marked paucity of framework breakage rate data for different species and size classes of fish, and a need for quantitative data on the grain-size fractions to which the eroded/broken framework contributes. For urchins, there are a number of known bioeroding species for which erosion rate data is necessarily based on substitute species rates. Addressing these gaps would be extremely useful. For both parrotfishes and urchins, information on the grain-size fractions to which faecal pellets contribute post-disaggregation is limited, and data on the constituent composition of these faecal pellets is sparse. Finally, for urchins, there is a pressing need to develop a methodology to support estimates of sediment inputs from urchin tests and spines, and ideally factoring for all urchins in a habitat and not just the bioeroding species. This has limited immediacy for our exemplar study sites as urchins are generally rare, but methodologies based around assessments of urchin test size as a function of known growth rates and test and spine CaCO3 content are currently being considered.
For the other major bioeroder group that generates sediment, the endolithic sponges, underpinning data to support calculations are sparse. There are a few experimental studies that provide community-level sponge erosion rate data (see ReefBudget webpage at https://geography.exeter.ac.uk/reefbudget/), but there is limited data on the rates at which different species erode substrate, and by extension the proportion of the eroded material that is lost to dissolution (but see Nava and Carballo, Reference Nava and Carballo2008; de Bakker et al., Reference de Bakker, Webb, van den Bogaart, van Heuven, Meesters and van Duyl2018). There is also limited data on the size fractions of the sediment that different sponge species generate, even though it is known that sponge ‘chips’ are common in fine grain-size fractions of reef sediments (Gischler et al., Reference Gischler, Dietrich, Harris, Webster and Ginsburg2013).
Methodologies for undertaking census-based assessments of sediment generation by calcareous green algal taxa do exist and are used within SedBudget (Perry et al., Reference Perry, Morgan and Salter2016, Reference Perry, Salter, Morgan and Harborne2019). However, the number of species for which the required underpinning metrics exists is low. Unless these same species occur at the site under investigation additional data to establish appropriate plant volume-carbonate content relationships, and plant sediment breakdown data should be collected, and can be readily obtained through localised collections (Perry et al., Reference Perry, Salter, Morgan and Harborne2019). Species turnover rate data is more involved and requires in situ staining and harvesting after growth (Mayakun et al., Reference Mayakun, Bunruk and Kongsaeng2014), but such additional data would be beneficial for many species. Similarly, location-specific collections and sample analysis to derive site-appropriate data for estimating epiphyte carbonate production from seagrass blades would be useful, as would data on plant turnover rates for all seagrass species from a wider range of localities.
As outlined in the methods, estimates of bivalve and gastropod carbonate production can presently be made based only on data for several common genera (Bosence, Reference Bosence1989). This is again an area where the potential for an expanded dataset exists, and one approach would be to analyse growth banding across a range of common species that could then be used to establish shell size-age relationships. These relationships can then be used in combination with shell CaCO3 weight data to refine the estimates of bivalve/gastropod production rates at the species (or at least genera) level as a function of shell length. Similarly, for benthic foraminifera the collection of test mass data to determine size-weight relationships, and of turnover rate data for a much wider range of genera and locations would be very useful.
There are thus a range of areas where additional datasets would help to improve sediment production rate estimates and support an improved understanding of variance in production rates across sites. Such data may inherently arise as wider applications of SedBudget are undertaken, because where possible, site-relevant data collection would clearly be advantageous. In cases of clear multi-species data gaps, these would lend themselves to more focused research. Our own work is starting to address some of these gaps, and it is additionally our intention to extend the methodology to capture a wider range of other sediment-generating taxa. This includes a wider range of calcareous green and articulated red calcareous algal (e.g., Amphiroa spp.), production associated with branched crustose coralline algae such as Lithothamnium spp., and sediment inputs from the breakdown of urchin tests and spines. Estimating sediment generation from these taxa is not currently possible due to the lack of calcification and turnover rate data. Further data is also needed to better constrain the proportions of different sediment constituent types where these derive from specific producers/processes, for example, from parrotfish and urchin erosion, and from seagrass epiphytes. Known existing proportional contribution data from these sources is provided at the bottom of the master summary sheet and applied to total production rates to estimate resultant sediment constituent yields. On-going work is looking to expand our knowledge of these processes and may ultimately support more refined modelling approaches to better predict production and variance therein from different species and processes. Given the importance of reef-derived sediment supply to nearby tropical shorelines, and the likelihood that the need to better quantify this supply will become increasingly important under both reef ecological change, and rising sea-level states, more extensive research on questions around reef sediment generation is urgently needed. SedBudget provides an opportunity to start to address these issues and we hope that the proposed methodology will act as a platform for work in this area.
Open peer review
To view the open peer review materials for this article, please visit http://doi.org/10.1017/cft.2023.14.
Supplementary material
The supplementary material for this article can be found at https://doi.org/10.1017/cft.2023.14.
Data availability statement
The authors confirm that the data supporting the findings of this study are available within the article and/or its Supplementary Material.
Acknowledgements
We thank the editors and two reviewers for their useful and supportive comments on the article, Didier de Bakker for thoughtful discussions on the developing methodology, and Aitana Gea Neuhaus and Sebastian Flotow for sample processing support.
Author contribution
C.T.P. initially devised the methodology, undertook field data collection and analysis and led the writing of the article. I.D.L. and M.S. both contributed to method development, field and lab data collection and analysis and writing and editing of the article.
Financial support
This work was supported by a Leverhulme Trust Research Fellowship (C.T.P., RF-2015-152), the Natural Environment Research Council (C.T.P., NE/K003143/1), and a Bertarelli Programme in Marine Science award to C.T.P. and I.D.L.
Competing interest
The authors declare no conflicts of interest.
Comments
This paper introduces a new census-based methodology called SedBudget that has been set up to address the challenge of how we can derive meaningful estimates of reef-derived sediment generation. This is an important challenge given the relevance of reef-derived sediment for the maintenance of many reef-fronted beaches and reef island systems. Based on a proposed set of consistent taxa-specific methodologies SedBudget generates estimates of total sediment production from reef taxa, and the types and size-class fractions of sediment generated. The paper provides an overview of the concept and approach used, and we illustrate the types of data the methodology can generate by applying the method to several reef sites in the northern Chagos Archipelago.
Underpinning the method are an extensive methodology handbook and three data entry spreadshhers (built in Excel to facilitate wide and easy access to the system). Post-review these will be made openly available on the webpage that hosts the sister methodology ReefBudget (at: https://geography.exeter.ac.uk/reefbudget/), but for the review phase these files have been made available at:
https://figshare.com/s/1c9df1482659469f8271
The data that SedBudget generates should not only have potential to support coastal sediment transport modelling studies through the acquisition of site-appropriate production estimates, but also to contribute to more integrated datasets capturing total site level biogenic carbonate production. This present iteration represents a first step in this important field of research.